It has been a while, but I promise I haven’t forgotten about the blog! College is an incredibly hectic time – there’s constantly problem sets due, midterms all the time (the excuse, “oh sorry, I can’t because I have a midterm soon” always works because you’ll probably always have a midterm or final within three weeks), and plus, of course, the random crazy adventures like running a 12K at 5 AM :). But, one of the things that has absolutely made up the core of my college experience and one of my favorite things about Stanford is my research. I’m super grateful to be working in the Bertozzi Lab under Dr. Carolyn Bertozzi. If you don’t know, Dr. Carolyn Bertozzi is the winner of the 2022 Nobel Prize in Chemistry along with Dr. Barry Sharpless and Dr. Morten Meldal for their work in bioorthogonal and click chemistry. And as I’m doing a click chemistry experiment next week at the lab, I’d like to introduce a bit about click chemistry, and what makes it so amazing!
What is click chemistry?
Synthesizing molecules is hard. Prior to click chemistry, the process for synthesizing molecules involved complex, multi-step processes with careful control over the reaction conditions and other factors, often also producing low yields. So in the early 2000s, American chemist Barry Sharpless discovered a way to “click” these molecules together like Legos, in a process that he coined as “click chemistry.” This reaction allows for molecules to be attached together in simple reaction conditions with few side reactions occurring, and does not react with oxygen or water. It also has very high yield, as Sharpless stated, “These reactions need to be perfect. In other words, they need to produce 99.999-plus percent yield. Two of them that we discovered in 2002 and 2013 nearly are.”
So, it’s hard to believe that you can go from a complicated chemical synthesis process into simply a few “clicks” to create molecules like Legos. But, here’s how it works.
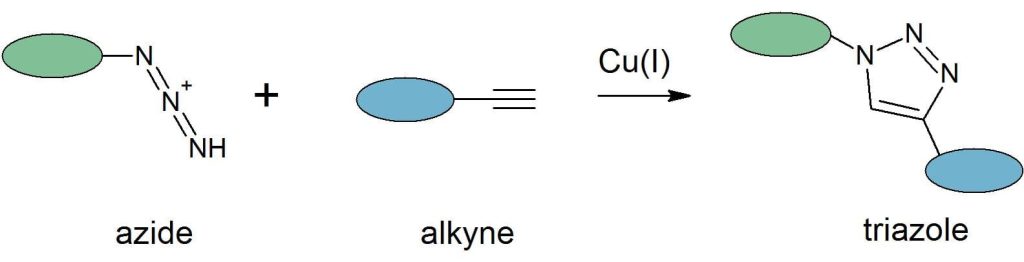
How does click chemistry work?
It starts with two molecules. One molecule has the azide, which is a molecule with a structure consisting of three nitrogen atoms – two with a negative charge and one with a positive charge, making it an anion. The other molecule is an alkyne, which is a chain of carbons and hydrogens known as a hydrocarbon that contains at least one triple bond. The azide and the alkyne molecules react together to form a triazole, which is a five-membered ring formed from the three nitrogen atoms and carbons. This process is known as a cycloaddition, which is when two molecules come together to form a cyclic ring. In the classic click reaction, the copper-catalyzed azide-alkyne cycloaddition (CuAAC), the cycloaddition is catalyzed by a copper molecule. Now, how does the copper work to catalyze the cycloaddition? The copper activates both the azide and the alkyne. The copper coordinates to the azide to increase the nucleophilicity – which just refers to the ability of a molecule or ion to donate an electron pair. In the alkyne, the copper coordinates to form a copper acetylide complex, making it into an electrophile – which is a molecule or ion that likes to form bonds with nucleophiles by taking up an electron pair. This causes the molecules to come together, where the nucleophilic terminal carbon of the alkyne will attack the electrophilic nitrogen in the azide to form a triazole ring.
Alright, that was a lot of chemistry. But in summary, there is a group of nitrogens – the azide, and a chain of carbons and hydrogens – the alkyne. With the addition of a catalyst, the two bond together to form a five-membered ring called a triazole. This reaction is highly selective, largely due to the selectivity copper has for the reactants and the inertness of the azide and the alkyne.
But the problem is, copper is highly toxic to living systems, which makes copper-catalyzed chemistry unsuitable for use in biological systems. And of course, chemistry often has important applications in biology, which makes it incredibly helpful to be able to do click chemistry in living systems. So, here’s where Dr. Carolyn Bertozzi’s work comes in.
Dr. Carolyn Bertozzi’s Work: Bioorthogonal Click Chemistry
Dr. Bertozzi is most known for her work in the field of bioorthogonal chemistry, which simply refers to chemical reactions that can occur in biological systems without causing other side reactions. This is incredibly important for the field of chemical biology, as it’s relevant in drug development, antibody-drug conjugation, drug delivery, and much more. So, the problem facing click chemistry was that copper is toxic to living systems. Because of this, Dr. Bertozzi, along with her graduate student, Eliana Saxon, came up with a solution through an existing reaction known as the Staudinger ligation. Instead of reacting the azide with an alkyne using copper, the bioorthogonal click chemistry reaction uses the Staudinger ligation to react the azide with a phosphine to produce an azalyide. However, the problem is that the product of the reaction, the azalyides, are not stable in water. Because of this, the Bertozzi group found a solution by placing an electrophilic trap, in particular, a methyl ester, in the phosphine to capture the nucleophilic azalyide in an amide bond. This amide bond is more stable compared to the azalyide that usually gets broken down in water, therefore allowing for a stable reaction that does not cause side reactions, as the phosphine and azide are both unreactive to other biomolecules.
So, what are the applications for click chemistry?
The ability to attach molecules together to synthesize compounds is an incredibly important discovery that has lots of applications! For example, click chemistry is often used in bioconjugation, drug discovery, probes for cellular localization, and development of other chemical tools. In one of the first experiments that Dr. Bertozzi used to show the uses of bioorthogonal chemistry, she was able to label the complex sugars on the cell surface with markers, even imaging a live zebrafish embryo by using click chemistry to attach a probe to the cell surface sugars, known as glycans.
One major use case for click chemistry is for bioconjugation, which is an experiment that I’m excited to be doing in the upcoming weeks! The experiment I’m doing is similar to the experimental protocol for antibody-drug conjugates. It involves conjugating a monoclonal antibody to an agonist drug. I will be coupling NHS, N-Hydroxysuccinimide, which contains an azide, to the amino acid, lysine, the antibody, which will allow it to be attached to the alkyne that is conjugated to the ligand we hope to bind the antibody to. Through click chemistry, we are able to conjugate different things together, which is incredibly useful in the lab!
Anyways, that’s my quick recap of click chemistry! It’s one of my favorite topics now, and I’m super excited for this opportunity to be working on this in the lab. What do you think?
Bibliography
A New Way To Engineer Cells: The Staudinger Ligation. https://www2.lbl.gov/Science-Articles/Archive/staudinger-ligation.html. Accessed 2 June 2024.
Agarwal, Paresh, et al. “Systemic Fluorescence Imaging of Zebrafish Glycans with Bioorthogonal Chemistry.” Angewandte Chemie (International Ed. in English), vol. 54, no. 39, Sept. 2015, pp. 11504–10. PubMed Central, https://doi.org/10.1002/anie.201504249.
“Click Chemistry.” Scripps Research Magazine, https://magazine.scripps.edu/features/2019/spring-summer/click-chemistry/. Accessed 2 June 2024.
Hein, Jason E., and Valery V. Fokin. “Copper-Catalyzed Azide–Alkyne Cycloaddition (CuAAC) and beyond: New Reactivity of Copper(i) Acetylides.” Chemical Society Reviews, vol. 39, no. 4, Apr. 2010, pp. 1302–15. PubMed Central, https://doi.org/10.1039/b904091a.
Meldal, Morten, and Christian Wenzel Tornøe. “Cu-Catalyzed Azide−Alkyne Cycloaddition.” Chemical Reviews, vol. 108, no. 8, Aug. 2008, pp. 2952–3015. DOI.org (Crossref), https://doi.org/10.1021/cr0783479.Saxon, Eliana, and Carolyn R. Bertozzi. “Cell Surface Engineering by a Modified Staudinger Reaction.” Science, vol. 287, no. 5460, Mar. 2000, pp. 2007–10. DOI.org (Crossref), https://doi.org/10.1126/science.287.5460.2007.